ITER project
The ITER (“The Way” in Latin) facility is an international research project that is being constructed in Cadarache, France. The terms and conditions of this collaborative effort are provided in the Agreement on the Establishment of the ITER International Fusion Energy Organization for the Joint Implementation of the ITER Project (ITER Agreement) dated November 21, 2006. The seven ITER partners are China, Republic of Korea, Japan, the European Union (host party), the Russian Federation, India, and the United States
Like many of its fission counterparts, a fusion reactor will employ light water (i.e., H2O) to transfer heat from the Tokamak (i.e., plasma) to a boiler to produce the steam that will then drive conventional steam turbines to produce electrical power. While ITER is not designed to produce electricity on a commercial scale but, instead, is intended to demonstrate sustained plasma burn and hence the feasibility of fusion power, it too will be cooled by light water.
The US is responsible for the design, fabrication, acceptance testing, and delivery of the Tokamak Cooling Water System (TCWS). The largest and most complex loop of TCWS is IBED PHTS (Integrated First Wall/Blanket/ Edge Localized Mode and Vertical Stabilization Coil/Divertor Primary Heat Transport System), which is designed to remove 880 MW of heat from different in-vessel components. IBED PHTS chemistry is controlled by the Chemical and Volume Control System (CVCS), which includes mechanical filtration, ion exchange, and degassing. It provides control of radiolysis and radiolytic products, among other functions.
OLI’s model for predicting radiolysis and corrosion damage in ITER
The role of OLI Systems in the current project is in estimation (modelling) of the concentrations of all species at any location of the coolant circuit that in turn allow to estimate electrochemical corrosion potential (ECP) at any point in the loop as a function of time and finally to estimate possible corrosion damage, OLI Systems was chosen and certified by Oak Ridge National Laboratory (ORNL) to accomplish this task due to its proven history in modelling complicated chemical and electrochemical systems and developing reliable scientific simulation software. The information about concentrations can be obtained by solving the equations of mass balance for all species, if the fluid conditions (e.g., temperature, radiation dose rate, hydrodynamics) are known at every point in the loop.
For the assessment of the behavior of the radiolysis species in the entire IBED PHTS, this system can be schematically represented with two parts: Plasma Flux Area (red) and Out-of-Flux Area (yellow), as shown in Figure 1.
In reality, for the analysis of the ITER IBED PHTS, the Plasma Flux Area (PFA) area can be subdivided into modules and blocks and, within each block, the known geometrical parameters (i.e., volume, length, diameter) and environmental conditions (i.e., temperature, flow rate, γ-photon and neutron energy deposition rates) are deemed to be constant during the burn and dwell periods. The entire PFA is represented as a series of combinations of these blocks (with the same mass rate of water), by analogy with electric circuits. Each independent series of block combinations is called a “module.” Within each module, the mass flow rate is constant. The IBED PHTS in the Plasma Flux Area (subject to intense gamma and neutron radiation) comprises 12 independent modules, each containing from 1 to 19 blocks. The Out-of-Flux Area (yellow) where energy deposition rate is negligible is considered formally as a single block. The real block diagram of IBED PHTS can be found in Refs. 1 and 2.
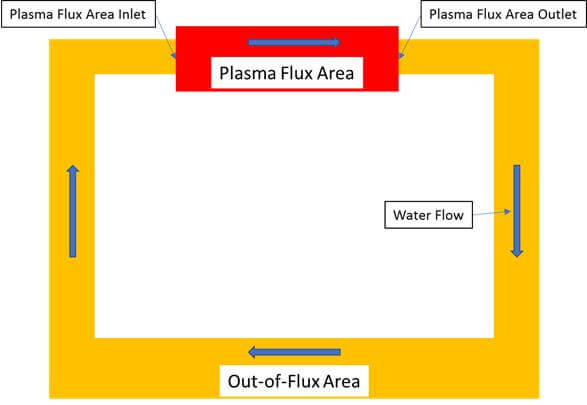
Interaction of the ionizing radiation with the coolant (water) results in the generation of a variety of radiolysis products: , H, OH, H2O2, HO2, ,O2, , ,O–, O, H2, OH–, H+, that are included into the model. The presence of the following metal ions: Cu+, Cu2+, Fe2+, Fe3+, in the solution is also considered. These ions may act as homogeneous catalysts for some of the reactions in the radiolysis model presented in [1, 2]]. In addition, it is assumed that, because of the influence of radiation on N2 molecules and due to the generation of nitrogen species via the nuclear reactions 16O8(1n0,1p1)16N7 and 17O8(1n0,1p1)17N7 with the oxygen isotopes in water, the following species are present in the solution:
.
The full list of 89 chemical reactions included into the model can be found in [1, 2]. In accordance with our knowledge OLI’s model is the most comprehensive model of radiolysis in ITER from the point of view of the number of species and chemical reactions included into the consideration. It is also important to note that the OLI model describes concentrations of species not in a single location (as it was performed in other models) but simultaneously in all blocks of IBED PHTS. Practically, the output concentrations in each block are considered as input concentrations for the consecutive block. Accordingly, the OLI model describes radiolysis in TOKAMAK cooling water system under real operation conditions. l
Results of modeling
It must be noted that from an electrochemical/corrosion viewpoint, there is no pressing need for knowing the concentrations of many of the highly energetic species. This is because the contribution that any given species makes to determining the electrochemical potential and the corrosion rate is roughly proportional to its concentration, since rate of transport to the surface and the partial current density are often mass transport controlled and hence are proportional to the concentration. Calculation (performed by using the model described above) show that the most dangerous source of corrosion of the IBED PHTS material are oxidizing species such as O2 and H2O2, For this reason large amount of hydrogen is added to Pressurized Water Reactor (PWR) primary coolant circuits and Boiling Water Reactor (BWR) cooling loops (operating under Hydrogen Water Chemistry) to suppress the radiolytic generation of oxidizing species and/or to displace the corrosion potential of structural components in the coolant circuits in the negative direction.
Below, Figure 2 shows the concentrations of O2 and H2O2, inside the block (Equatorial Port) with the most severe operating conditions (high neutron and γ deposition rates) as a function of the initial hydrogen concentration in the system.
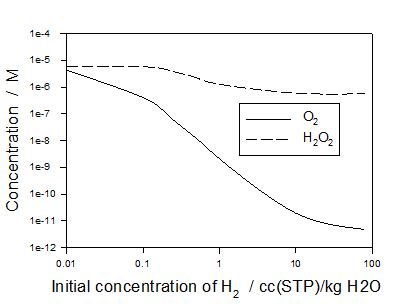
As we can see the addition of hydrogen is very effective for suppressing oxygen but much less effective for suppressing hydrogen peroxide under conditions inside IBED PHTS of ITER,
On the other hand, calculations show that even small amount of initial concentration of hydrogen (1 cc (STP/kg H2O) leads to the stabilization of the concentrations of the radiolysis species that takes place after the first revolution cycle (see Figure 3). This means that there is no accumulation of radiolysis products in the Out-of-Plasma zone in IBED PHTS if certain amount of hydrogen is injected into the water. The concentrations marked by cycles = 0 in Figure 3 correspond to the initial conditions in the IBED PHTS.
Calculations also show that Cu and Fe are predicted to be in their lower valence form in the IBED PHTS. If the concentrations of these ions do not increase above 10 ppb, these ions will have no significant influence on the radiolysis of water in the IBED PHTS. However, the presence of Cu and Fe in the solution might affect the rates of heterogeneous reactions that influence corrosion processes.
By using obtained concentrations of species the estimation of corrosion rates, corrosion potentials and repassivation potentials were performed by using OLI models and software [3, 4]. Calculations show that general corrosion is very low and offers no significant threat to the integrity of IBED PHTS. Calculations also show that repassivation potentials in all blocks are much higher than corrosion potentials and, accordingly, the stable pitting corrosion is unlikely in the IBED PHTS.
You can get more information on OLI’s solutions for the power generation industry here. Email [email protected] or complete this form if you would like to engage with a technical expert at OLI Systems or have a sales person contact you.
References:
- D. D. Macdonald and G. R. Engelhardt, “Review and Assessment of Radiolysis in the TCWS IBED PHTS.” Prepared by OLI Systems, Inc. Cedar Knolls, NJ, under contract No. 6400014199 with Oak Ridge National Laboratory, US ITER, Oak Ridge, TN (2017).
- A. Petrov, D. Macdonald, and G. Engelhardt, “Assessment of Radiolysis in Tokamak Cooling Water System of ITER Fusion Reactor”, Proceedings of the 21st International Conference on Water Chemistry in Nuclear Reactor Systems, September 9 – 14, 2018, San-Francisco, CA. EPRI, Palo Alto, CA, 2019: 3002016101.
- A. Anderko, “Modeling of Aqueous Corrosion,” in Shreir’s Corrosion, ed. T.J.A. Richardson (Amsterdam, Netherlands: Elsevier, 2010), pp. 1585 – 1629.
- N. Sridhar, C.S. Brossia, D.S. Dunn and A. Anderko, “Predicting Localized Corrosion in Seawater” Corrosion, 60 (2004) 915-936.
- D. D. Macdonald, G. R. Engelhardt, and A. Petrov, “A Critical Review Of Radiolysis Issues In Water-Cooled Fission And Fusion Reactors: Part II, Application In Predicting Corrosion Damage”, in Corrosion and Materials Degradation Under Irradiation: From Understanding To Mitigation…, Edit. B. Normand, 2021 (in preparation).